by Ishaan Goel
Hydrogen has become increasingly prominent as a potential carbon-free fuel, for both automobiles and providing electricity to buildings. It has direct applications in decarbonizing important industries like steel, and can serve as a storage medium for extra renewable energy over seasonal durations too.
Since hydrogen gas does not occur naturally in our atmosphere, its method of production is an essential component of the hydrogen economy. There are several such methods (discussed in detail here), but the one with least emissions involves using renewable power to run electrolyzers – devices that use electricity to convert water into hydrogen and oxygen gas.
This article introduces a series on electrolyzers which will explore the various technologies, the efforts and challenges to improving them, and their prospects for wide-spread adoption.
While there exist several types of electrolyzers, the two primary kinds today are alkaline electrolyzers (ALKE) and polymer electrolyte membrane electrolyzers (PEME).
ALKALINE ELECTROLYZERS
ALKEs comprise two solid electrodes containing catalysts, immersed in a liquid, alkaline electrolyte. An electric current passes through these electrodes, which splits water at the negative electrode into hydrogen gas and hydroxide (OH–) ions. The positive electrode attracts the hydroxide ions, which travel to it within the electrolyte. They combine there to form oxygen gas and electrons, which keeps the reaction going. During this process, they pass through a porous membrane (diaphragm) designed to internally segregate the gases.
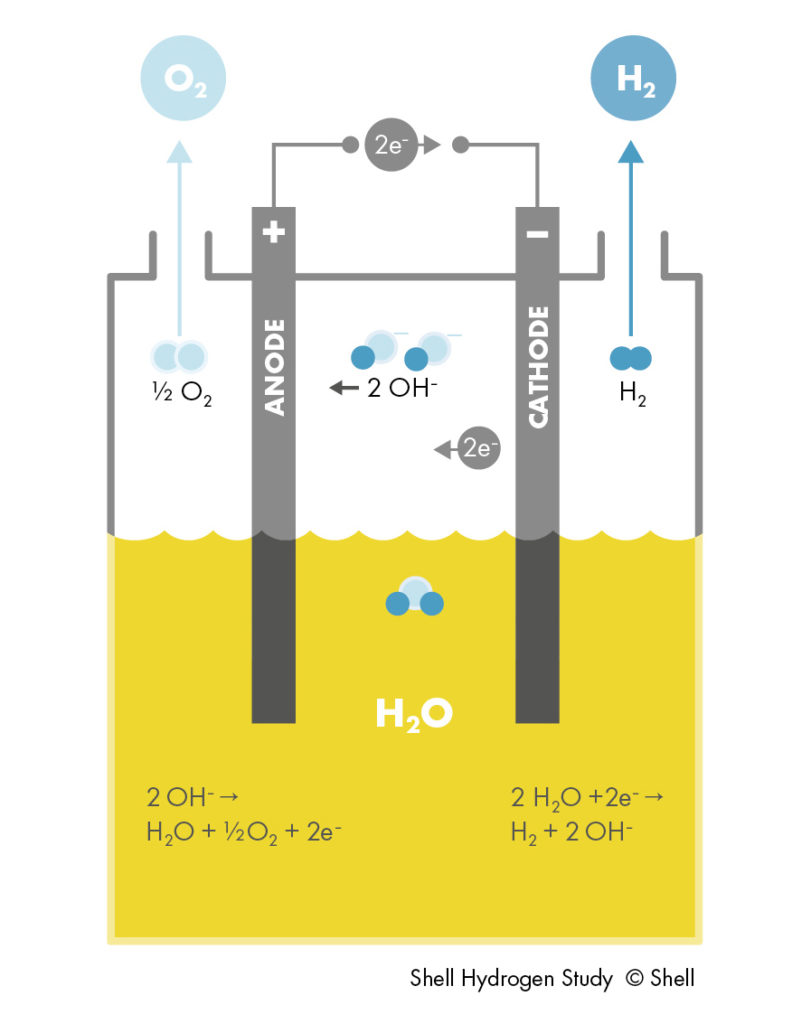
PEM ELECTROLYZERS
Instead of liquid electrolytes, PEMEs utilize solid polymer compounds present within a porous, central layer. This layer is surrounded on either side by electrodes with catalysts, followed by meshy gas diffusion layers, and then by bipolar plates. Water (as steam) is introduced through the bipolar plate, and passes through the diffusion layers towards the positive electrode. Here, it splits into oxygen gas and hydrogen (H+) ions. The ions permeate through the central electrolyte towards the negative electrode, where they combine with free electrons to form hydrogen gas that is collected through the other bipolar plate. In both electrolyzer variants, the catalysts hasten the reaction.
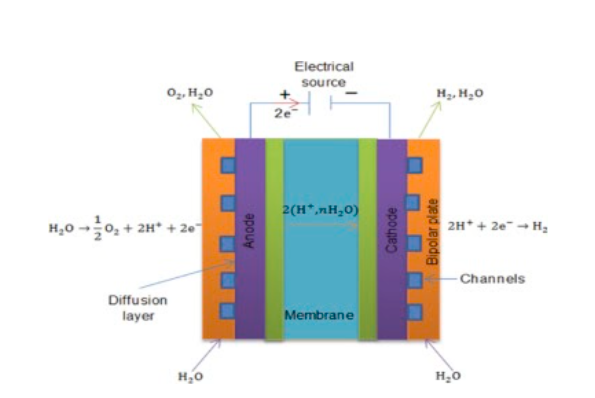
ADVANTAGES OF PEMEs
Each technology comes with its own tradeoffs. PEMEs are advantageous because their production level responds faster to changes in power supply and they can tolerate higher current densities, making them ideal for fluctuating power sources like solar/wind energy. The layered structure and lack of liquid electrolyte allows for compact designs, so they are effective within space constraints. The solid electrolyte enhances ion conductivity, which boosts the overall efficiency. Any electrical energy lost as heat can be redirected into the conversion of water into steam, which reduces wastage and further increases efficiency.
WEIGHING UP ALKEs
However, PEMEs have higher capital costs than ALKEs. Their conductivity is highly dependent on factors like hydration of the membrane and temperature, and maintaining optimal operational conditions requires extra components. They also need platinum and iridium-based catalysts, which are not only very expensive, but also limit the scalability of PEMEs based on their availability.
ALKEs usually use cheaper nickel or stainless steel-based catalysts. Further, their durability will also be higher because of less corrosive environments and the replaceability of the electrolyte after extensive usage. ALKEs also tend to exhibit lower internal mixing of gases, so the produced hydrogen has greater purity. However, nickel-based membranes and separators are being developed for PEMEs too, which may possibly bring down the cost differential in the future.
OTHER ELECTROLYZER TYPES
To blend the best of both technologies, certain firms have come up with anion exchange membrane electrolyzers (AEMEs). These utilize solid membranes similar to PEMEs, but involve the transfer of hydroxide ions instead of hydrogen ions like ALKEs. As a result, the catalyst can be cheaper nickel or stainless steel instead of platinum/iridium while still preserving efficiency and responsiveness benefits. Companies actively engaged in the area include Enapter and Evonik Industries (EVK.DE, EVKIF, EVKIY). Another alternative is ThyssenKrup’s (TKA.DE, TYEKF, TKAMY) “advanced alkaline electrolysis”, that aims to deliver modules with lower maintenance and capital costs.
DESIGN INNOVATIONS
Electrolyzers are usually sold as modular “stacks”, which consist of multiple units conjoined together to scale up their joint output. For instance, NEL Hydrogen (D7G.F, NLLSF, NLLSY) produces electrolyzer stacks ranging in production capacity from 1.05-3880 nm3/hour, suitable for large-scale centralized production or household/office-level distributed applications. Some firms also design for the produced hydrogen to have pressures suitable for direct use – for example, Giner ELX (a subsidiary of Plug Power (PLUG)) systems yield hydrogen directly in an ideal range of about 435-580 psi.
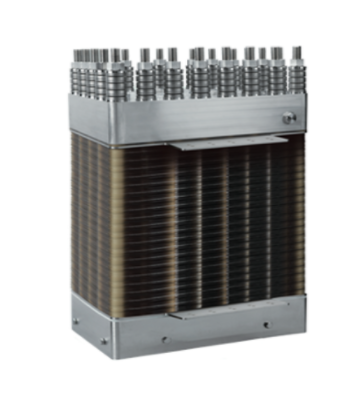
CONCLUSION
With growing interest in the adoption of hydrogen, active research continues into improving existing electrolyzer technologies across various parameters. The next articles in this series will focus on the current status of such parameters, including capital costs, for both ALKEs and PEMEs.
Disclosure: The author has no interests in any firms mentioned.
***